Africa’s Living Rivers: Managing for Sustainability
Africa’s human population is growing rapidly and is set to account for 40 percent of global numbers by 2100. Further development of its inland waters, to enhance water and energy security, is inevitable. Will it follow the development pathway of industrialized countries, often destructive of ecosystems, biodiversity, and river-dependent social structures, or can it chart a new way into the future based on global lessons of equity and sustainability? This essay tracks the global and African growth of the benefits and costs of water resource developments, explores the reasons for the costs, and offers insights on new scientific thinking that can help guide Africa to a more sustainable future.
Fresh water is a seriously limited and limiting resource. Of the 1.4 billion cubic kilometers of water on Earth, 97 percent is seawater with only limited potential for terrestrial use.1 Two-thirds of the remainder is locked in ice caps and glaciers, and one-third is in liquid form, with most of this stored deep below the earth’s surface in aquifers. The remaining liquid fraction, not much more than 200,000 cubic kilometers (0.014 percent of all water on Earth), is stored in freshwater ecosystems that occupy less than 1 percent of the earth’s surface: rivers, lakes, deltas, floodplains, peatlands, swamps, lagoons, pans, bogs, seeps, and estuaries, among others.2
Most life on Earth depends on these inland waters, but they are degrading faster than any other kind of ecosystem due to the unprecedented scale of human interventions. As degradation continues and accelerates, their ability to support human endeavor falters and, in all too many cases, fails. In this century, there is a deepening understanding of the implications of this, and of our need to live in harmony with the natural world. Nowhere is this more important than for Earth’s inland waters. This essay provides a global and African view of how and why they have degraded and offers a Southern African perspective on one way in which we could do better.
The world population stood at five million people in 8000 BC, one billion in 1800, and seven and a half billion in 2017. The UN predicts it will reach eleven billion by 2100 in what appears to be a slightly flattening trajectory.3 From 1970 to today, there has been a doubling of the world’s human population, a fourfold increase in the global economy, and a tenfold increase in trade.4 All of this depends on fresh water. Africa, with about 9 percent of the world’s freshwater resources, is a prominent part of this growth. It is a low- to medium-income continent with six of the world’s fastest-growing economies.5 Its human population, presently 1.4 billion or 17 percent of global numbers, is predicted to reach 4.5 billion by 2100 (40 percent of global). Water resource development to meet this growth is inevitable and will take place under the scrutiny of Sustainable Development Goal 6 (SDG 6: clean water and sanitation) and SDG 15, which weakly addresses inland waters as a subsection of life on land. Moving along the development pathway a few decades behind more industrialized economies, Africa will face, perhaps more than any other region, a challenge to develop and manage its water resources without repeating the mistakes made by others.6 Can it learn from the past and proceed more carefully?
Water resource developments were originally designed to provide water as and where people needed it, and much of humanity has benefited from that. The middle to late-1900s was a great era of dam-building, leading globally to about 58,000 very large dams and hundreds of thousands of smaller ones providing better sanitation; water on tap in homes, gardens, and businesses; and food security from irrigated crops.7 Even with this scale of construction, two-thirds of the world’s people presently face water shortages for at least one month a year, and almost two billion people live in areas at risk from severe water scarcity.8 Within Africa, sub-Saharan Africa, in particular, suffers from chronically overburdened water systems through increasing stress from fast-growing urban areas.9
There is presently a second major surge in dam-building, this time to generate electricity.10 Hydropower accounts for a significant and growing proportion of the electricity generated in Eastern and Southern Africa, representing, for instance, 90 percent of the existing supply in Ethiopia, Malawi, Mozambique, Namibia, and Zambia.11 The Programme for Infrastructure Development in Africa estimates that total energy generation for the continent will need to continue to increase by 6 percent per year for the next few decades, and that large hydropower dams planned or under construction in Southern and Eastern Africa could double the present generating capacity. The pattern is mirrored globally, and clearly much more will be done to secure water, food, and energy for people, but at what cost?
That cost comes mainly in the form of the degradation of the donor river systems, a severe decline in species and habitat biodiversity, and impacts on the lives and livelihoods of those depending on healthy rivers. Rivers are living ecosystems that provide a range of services that we all benefit from: delivery of freshwater over vast landscapes; purification of used water; attenuation of floods by storage in floodplains; replenishment of groundwater that in turn supplies river flow in the dry season; stabilization of banks and coastlines; sequestration of carbon in wetlands, thereby helping to keep the brakes on climate change; and supporting the life stages of a myriad of plants and animals. Through their natural functioning, rivers support hundreds of millions of people who live along their banks and depend on them directly for food, medicinal herbs, construction materials, firewood, grazing, and shelter, as well as those further away who also depend on them for resources such as fish. They are highly valued in human cultures through recreation; national symbols and borders; religious and spiritual upliftment; and inspiration for books, music, art, and photography. They are one of Earth’s most valued and important ecosystems, and arguably the most vulnerable.
Rivers are now severely at risk from the scale of water resource development and dam-building already completed and presently underway. The diversity, health, and resilience of whole river systems are being detrimentally affected, with impacts spreading downstream, across national boundaries, and into oceans.12 The likelihood of substantial knock-on effects into politics and human conflict is increasing. What exactly is happening?
Dams and abstractions of water from rivers alter the pattern of their flows to achieve benefits for people–that is their purpose. All dams fragment river systems, and all flow manipulations and water abstractions affect river ecosystems to greater or lesser extents. The more we intervene, the more rivers change, and different kinds of interventions alter them in different ways as the following few examples illustrate. A large dam that stores floods could stop downstream floodplains from flooding, with the loss of habitat and grazing for wildlife and livestock. On Zambia’s Kafue Flats, for instance, the number of Kafue Lechwe (an antelope that is endemic to the Flats) declined from 110,000 in 1973 to fewer than 30,000 in 2015 as a result of, inter alia, reduced flood releases from the upstream Itezhi-Tezhi Dam.13 This is also of concern for Zambia’s national herd, 20 percent of which is supported by the Kafue Flats. Reduced flooding of floodplains around other African river systems has resulted in a decrease in groundwater recharge and an increase in hot, dry-season wildfires.14
Dam walls stop upstream and downstream movement of species, such as fish moving upstream to spawning sites. If the fish do not reach the spawning sites, they do not produce the next generation of fish and so their numbers decline. Water released from dams in the dry season can wash away downstream plants and animals not adapted to higher-than-normal flows, such as juvenile fish, thereby disrupting their life cycles and reducing the number of fish.15 A reservoir kilometers long is as much a barrier as the dam wall creating it, and equipping dams with fish ladders to move the fish upstream past the dam wall may be unsuccessful, as fish become disorientated by the lack of flow in the still waters of the reservoir and may never find the upstream river to their spawning grounds.16
Releasing an even flow of water from a dam throughout the year can provide optimal conditions for some species to increase to pest proportions. Outbreaks of blackfly in dammed stretches of the Orange River in South Africa, for instance, affected livestock so seriously that losses of up to US$2.5 million per annum were experienced by farmers along one short section of the river as the flies attacked the cows and milk production failed.17
Dams hold back sediments moving down the river, leading to a loss in storage capacity of the reservoir, with more than 0.5 percent of the global volume available for water storage in reservoirs lost annually as sediments settle behind dam walls.18 The dams cause loss of riverine habitats and species as sediment-hungry water released from them erodes the downstream riverbed and banks.19 Such a change in sediment supply can also result in adjustments of the downstream channel, affecting country boundaries, such as occurred with the Ruo River on the border between Malawi and Mozambique and the Usuthu River between Mozambique and South Africa.20
Peaking hydropower dams may abnormally and massively increase, in a single day, the range of flows experienced in the downstream river, washing away downstream habitats, plants, and animals. Hydropower dams are not green energy.21 Like all dams, they disconnect the flow of water, sediments, and life along rivers, degrading habitats, blocking migratory fish, and reducing gene pools and new generations of aquatic life.22
Many dams are in areas relatively untouched by development until the last decade or so, targeting remote areas and river systems that supply water, food, and lifestyle support to local riparian people as well as orders of magnitude more people whose livelihoods depend on the downstream rivers, estuaries, and near-shore marine areas. These people rarely had, and many still do not have, a voice in the decisions regarding the development of their rivers, and commonly do not benefit from them.23
Africa has a goodly share of large dams, most triggering widespread ecological and social concerns. For example, Kariba Dam, built in the 1960–1970s, controls 90 percent of the total runoff of the Zambezi River. It created the world’s largest man-made reservoir but is now operating below par due to droughts and instabilities of the dam wall. Before construction, there was “barely any assessment of the potential ecological impact of the dam, much less the human costs.”24 Downstream of Kariba, Cahora Bassa Dam, also built on the Zambezi in the 1970s, was seen by scientists at the time as the least-studied and possibly least environmentally acceptable dam project in Africa.25 Presently under construction, the multi-billion-dollar Grand Ethiopian Renaissance Dam on the Blue Nile has triggered intense nationalistic fervor in both the upstream (damming) and downstream (impacted) countries (see Harry Verhoeven’s essay in this issue of Dædalus).26 Analyses of any ecological and social-impact assessments of the dam that may or may not have happened are completely overshadowed by political announcements. The Grand Inga Dam, on the Congo River in the Democratic Republic of Congo, is located on the continent’s second-largest river by length and the world’s second-largest river by volume. Coming with an eye-watering price tag of more than US$80 billion (including for transmission lines), it is a series of six dams: two now completed, one in the design phase, and the later ones dependent on available funding and markets. It could become the largest power station in the world, producing 40,000 megawatts of electricity, more than twice as much as Three Gorges Dam on the Yangtze in China, and perhaps providing more than one-third of all electricity produced in Africa. It has proceeded without any risk information being made public and with no major environmental or social studies as of 2019.27
From little boys kept out of school to stop livestock wandering across a de-watered river28 to an increase in waterborne diseases, from the collapse of a coastal prawn industry to the loss of clean drinking water, the list of the negative side of development continues to lengthen.29 The details usually differ from river to river, and so understanding of what could happen is increasing slowly, one river at a time.
As flows, sediment loads, and species have been affected, rivers have also universally been used as disposal units for domestic and industrial waste; their sediments have been mined at industrial levels, destroying habitats for fish and other life forms; they have been contaminated with agricultural fertilizers and pesticides, reducing water quality that then needs costly purification; exotic species have been introduced that cause declines in native species and loss of ecosystem services; aquatic life has been overharvested so that stocks decline; and floodplains and banks that are an essential part of a river’s continued healthy functioning have been encroached upon.30
As a result, rivers and other inland waters are vanishing or drastically degrading globally at a higher rate than any other kind of ecosystem–three times faster than forests, for instance.31 The implications are profound for climate change, as these ecosystems are one of the most important tools in counteracting climate change, storing about three times as much carbon as other landscapes.32 Equally disturbing are the trends in species numbers. The 944 aquatic species assessed by the World Wide Fund for Nature (WWF) for its 2020 Living Planet Index have declined by an average of 84 percent, or 4 percent per year, since 1970, with one-third now threatened with extinction.33 Across all land and ocean ecosystems, Africa recorded the second-highest level of biodiversity decline (65 percent) after Latin America and the Caribbean (94 percent), with habitat loss and overexploitation of species being the main drivers of decline. We would do well to heed the words of biologist Edward O. Wilson on the importance of biodiversity: “This is the assembly of life that took a billion years to evolve. It has eaten the storms–folded them into its genes–and created the world that has created us. It holds the world steady.”34
Humans are changing the world’s limnological landscape and the planet at an unprecedented and increasing rate; the world is indeed becoming more unstable. Extreme events are increasing in number–floods, droughts, pandemics–and the present trajectory offers few solutions for sustainability. Climate change is adding a new layer of uncertainty and could further accelerate the degradation of the last half-century, but the details are uncertain.35 In recent work on the Okavango River System in Southern Africa, for instance, predictions from thirty-six global climate models can be divided into two main groups: one predicts a wetter, hotter future for the basin and the other a drier, cooler future.36
What has gone wrong? How has the laudatory work to provide water, food, and energy for all resulted in so much damage? We understand now that past water resource developments were designed, and decisions on development made, on engineering and economic criteria, prioritizing a narrow range of economic benefits.37 Up to about the end of the last century, little or no consideration was given by most governments, developers, or funders to the ecosystems that provided the water: their nature, plants, and animals, and the myriad benefits they provided to society. The mindset was one of exploitation of an available resource; the drive was to meet the ever-increasing demand for water, food, and energy with little understanding or thought of the interconnectedness of natural systems and our dependence on them. Proclaimed costs of the developments tended to be limited to those linked to construction and operation of the infrastructure, providing highly attractive options focused on benefits such as increased food, jobs, and growth. But these attractive, cheap options were only cheap because the social, ecological, and physicochemical costs of degrading landscapes and river systems were ignored. Such costs tended to be the outcomes of water resource decisions rather than inputs to them, and they were often unexpected and distressing.
Why were the outcomes unexpected? Before the second half of the last century, ecological and social specialists tended not to be employed in major water resource management arenas. Most were working in academia and research institutions isolated from water-development decision-making processes. Ecologists did not have the equivalent of the hydrological, hydraulic, and economic models available to engineers and economists, and were not taught how to create and use such models. They did not have experience with the power of models or training to create detailed predictions of possible futures (scenarios). Social specialists did not receive descriptions of how river systems could change to help them understand the social implications and formulate their responses to development proposals. Without such contributions, decisions on dams and other major water developments were being made by “far too few for far too many.”38
Thinking began to change as the global concern regarding the downside of the first era of massive water resource development become more vocal in the 1970–1980s, with popular articles such as “Damnation Comes to the Darling” and books such as When the Rivers Run Dry, Silenced Rivers, and Vanishing Waters.39 By the 1980s, river ecologists were shifting focus from species to ecosystems, acknowledging that species could not be conserved unless the environment that supported them was also conserved.40 They started to study how ecosystems functioned and to develop ecosystem models. By the 1990s, the new scientific discipline of Environmental Flows (EFlows) had emerged in response to global concern over dying rivers and was gaining international attention by guiding water managers on how much water should remain in rivers targeted for development.41
In 1997, the World Commission on Dams was formed to investigate the global ecological, social, and economic impacts of large dams. Its chair, Professor Kadar Asmal, wrote in 2000 that in the last century, we collectively “bought, on average, one large dam per day and there have been precious few, if any, comprehensive, independent analyses as to why dams came about, how dams perform over time, and whether we are getting a fair return from our $2 trillion investment.”42 The costs as well as the benefits were opening up to scrutiny.
From the turn of the century, this scrutiny has gathered pace and is upward and outward: moving past species conservation to focus on ecosystem management; from project-specific impacts on small sections of rivers, to the implications of developments for whole river basins and even the oceans they flow into; and from prescriptively recommending flows for a river, to a neutral approach of providing stakeholders with a range of potential future river conditions for their consideration.43 Scientists are describing more and more effectively how everything is connected and what could happen with management interventions. Their models can stand shoulder to shoulder with the engineering and economic ones to provide new kinds of information, and they are becoming part of water management and decision-making teams.
What do we know now that we did not know fifty years ago? What was learned along the way? We know that to keep rivers healthy, aspects of all parts of their flow regimes must be retained–floods as well as low flows–each in the season when it would naturally occur. An arbitrary single-number minimum-flow release from a dam to keep a river wet is convenient for planning and design purposes, but there is no evidence that it will support the river ecosystem. Indeed, the body of evidence indicates the opposite, with an allocation of a minimum flow to a river providing false comfort that “the environment is being taken care of” when in fact it is not.44
We know that experienced interdisciplinary teams are needed to populate the ecosystem models with data: hydraulicians; geomorphologists; fish, invertebrate, bird, reptile, amphibia, and mammal zoologists; botanists; aquatic chemists; microbiologists; climate-change modelers; and more. We know that the links to the social implications of a changing river need to be made, and so ecosystem models have to be extended to become ecosocial models that bring specialists in public and livestock health, social and cultural structures, resource economics, and more into the team.45 These teams can predict in detail how a river will change with water management plans and how this will affect the people dependent on its resources. Linked with the engineering and economic modeling, balanced descriptions of the predicted costs as well as the predicted benefits of a management plan are starting to replace the one-sided predictions of benefits from the past.
We know that the more interventions there are to natural rivers, the more their condition will change, increasingly affecting the huge numbers of people dependent on them. We understand that the choice of what that future condition should be is not a scientific one; there is no magic number that represents how much water to leave in a river in order to keep it healthy. Rather, it should be a decision of society, whereby stakeholders of all kinds from international to local groups are provided with understandable descriptions of possible futures, which they can then use to negotiate with their governments for the future they want for themselves and their children. In some cases, such as in national parks, it could lead to near-natural rivers retaining most of their flow; in other areas, where perhaps food production is a priority, it could lead to more water abstraction and rivers in consequently poorer condition, though not to the extent of becoming health hazards.
As Africa moves to further develop its water resources, it will be judged and hopefully guided by emerging thinking over the last decade on the need to adopt an ecosystem approach as explained by the Convention on Biological Diversity and Nature-Based Solutions.46 These approaches address societal challenges such as climate change and water and food security, but they also recognize the need to base human activities on a scientific understanding of the organization and functioning of the natural world in order to limit damage to the planet. Such an approach resonates with the mission statements, goals, and objectives of water authorities, which usually include mention of “sustainability” in some form but without specifying what is meant by the term or how it will be achieved.47 Common definitions of sustainable development along the lines of “to provide resources for present generations without compromising the needs of future generations” offer little practical guidance. How then do we measure success on the ground? It has been difficult in the past, a term easily used and then largely ignored, but the kinds of ecosocial models now available can help to put flesh on these aspirational bones.
South Africa has been a proud trailblazer since the 1980s of such thinking in the management of its water resources. It responded to the concern over its degrading rivers with early development of EFlows concepts and practices, and by writing protection of its inland waters into law.48 Other Southern African countries followed suit, some writing new legislation triggered by scientific work and others moving ahead of legislation to begin protecting their rivers.49
Differences emerged between the Southern African approaches to EFlows and that of more industrialized high-income countries.50 The latter had already developed most of their water resources, and their people are supported by a safety net of salaries and social payments. Much of their EFlow focus initially was on protecting valued species such as game fish, but more recently, they have turned to rehabilitation of their degraded rivers, with recommendations of EFlows and more to achieve this.51
Southern Africa, by contrast, has a wealth of natural resources still in near-natural ecological condition, and there is good awareness of the close links across vast landscapes between river condition and peoples’ livelihoods. Registering this and the emerging concern on degrading rivers, South Africa began the development of methods and concepts for guiding sustainable water resource developments in the late 1980s.52 These were along the lines of the ecosystem approach and nature-based solutions, although the terms themselves did not materialize for another decade or two.53
By 1992, South Africa had developed and was applying the world’s first holistic approach to determining flows for river maintenance: the Building Block Methodology (BBM).54 This was a prescriptive method that recommended amounts of water for river maintenance, but it could not respond to queries of what would happen to different aspects of the river system or to people if those amounts were not provided. The BBM provided the data that drove recognition of the environment as one of only two entities with a right to water in South Africa’s 1998 National Water Act, the other right being for basic human needs. All other uses of water in the country are controlled by permits, a situation now prevalent through most of Southern Africa. In adherence to the requirements of this act, a water allocation for ecosystem maintenance is now being determined for every major water course, wetland, estuary, and groundwater aquifer in South Africa, driven by a classification process that encompasses stakeholder input. Similar work is underway in other parts of the Southern African Development Community (SADC).55
By 2000, South Africa had moved on, developing and applying DRIFT (Downstream Response to Imposed Flow Transformations), one of the first ecosystem models to provide a neutral, interactive approach that predicts the impacts of a range of possible water developments on rivers and their dependent people, for consideration by stakeholders.56 Other kinds of predictive models are also being developed in South Africa and elsewhere, for example, in the United States, South America, Australia, Europe, and Asia.57 Some have a link through to the social implications. For simplicity, we focus here on DRIFT as an example of an African model whose developers determinedly set out to address the realities facing water scientists as they began to contribute to water resource management: sparse data (because new kinds of methods and models require new kinds of data, not just in Africa but worldwide); limited time and money; and a business-as-usual inertia among many dam-builders, international funders, and governments to engage meaningfully with new sustainability thinking.58
The shift to the neutral, interactive, scenario-building DRIFT model attracted the attention of the World Bank, Asian Development Bank, International Union for the Conservation of Nature, World Wide Fund for Nature, United Nations Development Programme, Food and Agriculture Organization, Deutsche Gesellschaft für Internationale Zusammenarbeit (German Corporation for International Cooperation), and a number of other organizations and governments in Africa and Asia, who moved to employ it. DRIFT has been used, for instance, to aid basin-wide planning for major transboundary rivers, including hydropower in the Lower Mekong River (Thailand, Lao People’s Democratic Republic, Cambodia, Vietnam); basin development of the Okavango River system (Angola, Namibia, Botswana); and resolution of a dam-related conflict in the Upper Indus River (Pakistan and India), where the Permanent Court of Arbitration in The Hague concluded that for an issue of the magnitude and importance of that under consideration, DRIFT was “an appropriate tool for estimating potential changes in the downstream environment.”59
In all cases, the outputs of the model predict in detail, for points along the river system, how the ecosystem would change and how people would be affected. This is done through use of a considerable list of indicators, each of which would change in a different way under each scenario. Physical indicators could include, for instance, depth of pools, bank erosion, extent of floodplain inundation, and aspects of water quality. Biological indicators could include individual species, such as tiger fish, fish eagle, elephant and Anopheles mosquitos; and groups of species, such as floodplain spawning fish, riparian trees, and much more. Social indicators could include household incomes, waterborne diseases, and access to potable water.60 Scenarios themselves can be chosen based on management proposals other than dams, to explore the implications of harvesting natural resources such as sand and fish; enhanced management of the catchment; and control of poaching.61 Climate change is now a common part of such investigations, usually done by running all scenarios to show the implications of proposed management actions, and then running them again with climate change superimposed, so that the difference caused by climate change alone can be estimated.62
At last, river managers, conservation agencies, riparian dwellers all along the system, and other stakeholders have the opportunity, through the range of models mentioned here and others, to obtain information that will help them understand the implications of a changing river, empowering them to voice their concerns or support. It has given decision-makers reason to pause and consider more comprehensively, eliciting quite profound feedback. A minister of the environment in Asia commented that he had never before understood the full implications of the decisions he made. A cabinet minister of a SADC country said, “We make decisions we think are right, only to discover later that some are disastrous. I could not sleep if I did not take every chance to improve our decision making.” A design engineer for a SADC dam said that the model outputs had helped them chart a new way forward, understanding crucial environmental issues and putting in place mitigation that resulted in no significant loss of planned hydropower generation. The CEO of a River Basin Organization commented that he now saw rivers in an entirely different way and could not go on as before.
What can decision-makers do with the new information from specialists and the new stakeholder inputs? It is their prerogative to make the decision on a water management proposal, but as stakeholders gain access to more information, the whole process becomes more complex while potentially more open and equitable. As part of this process, how do we unpack the mystery of sustainable use and ensure it is adhered to? Sustainable is an adjective for something that can be sustained, that is, something that is “bearable” or “capable of continuing at a certain level.”63 In this context, bearable suggests that it should be acceptable to people, and capable of continuing suggests that the natural inland waters that support humans should not be harmed to the point at which they can no longer do this. Thus, in water resource management, sustainable use can be seen as a trade-off that society negotiates and agrees on between what is gained and what is lost by development (or rehabilitation), a balance between water use and ecosystem conservation. The trade-off point could differ from basin to basin.
One approach that can aid this process is the concept of Development Space.64 For a river or basin, the Development Space is defined as the difference between current ecological and social conditions in the basin and the furthest level of development-driven degradation of the river found to be acceptable to governments and other stakeholders. Negotiating this end point helps them to identify their “mark in the sand”: the future that they do not wish for. This, in turn, makes obvious how much development potential is left in their basin.
In support of this endeavor, DRIFT can be set up with a series of development scenarios, each of which describes in detail a point along the development/degradation pathway. Negotiations between and within countries can then identify the furthest acceptable point of decline in an ecosystem and dependent social conditions across the basin: the point at which costs are perceived to outweigh the benefits of development.65 Once the Development Space has been agreed upon, negotiations can move on to how much of the space can be allocated to each country (in a transboundary river basin) or to each water-use sector (for a river within one country) (see Figure 1).
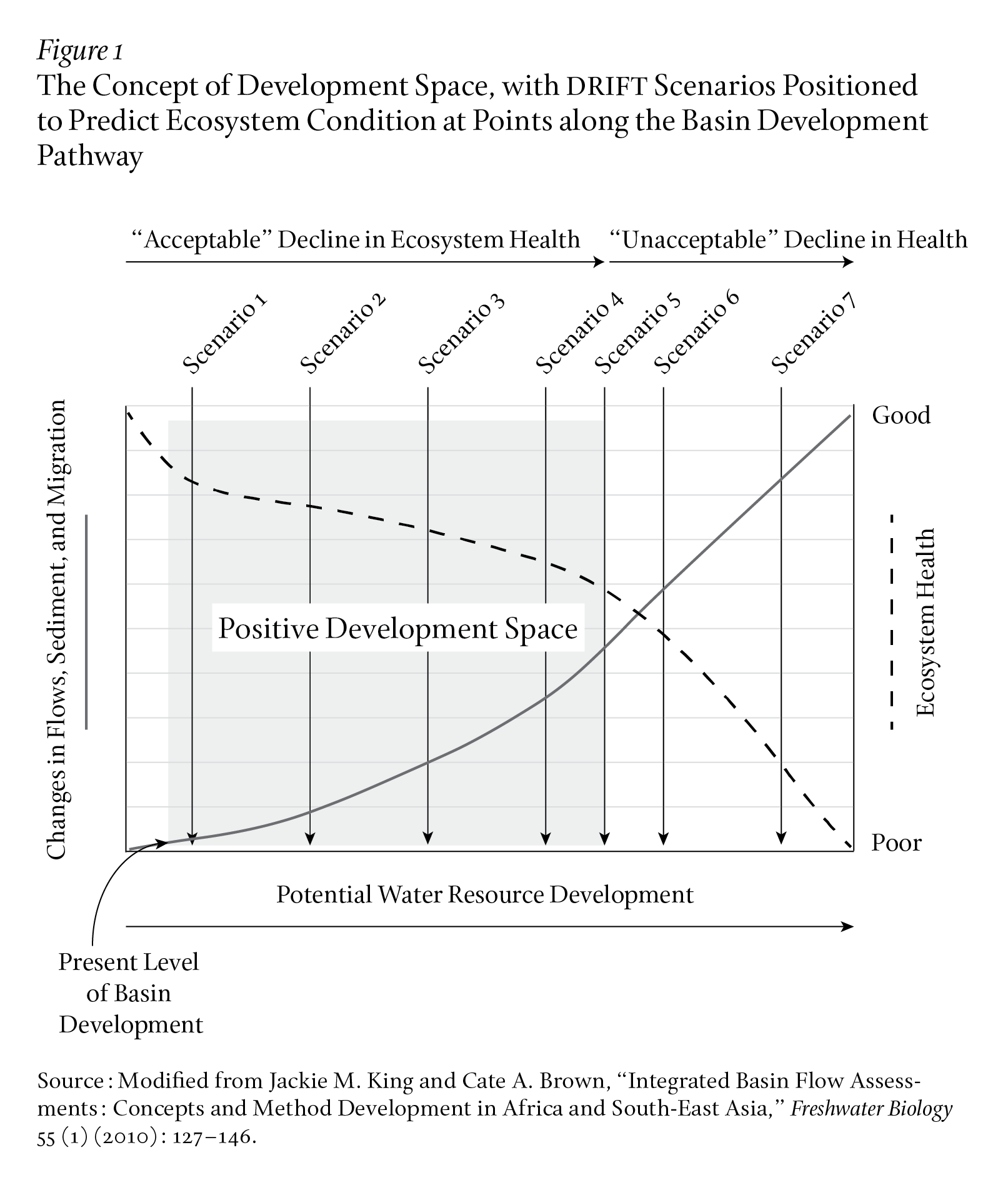
Where river systems are already heavily developed and degraded, the ecological and social consequences could be deemed now to be unacceptable, and the Development Space seen as negative. In such a situation, the same process could be used in reverse to plan what level of rehabilitation would be an acceptable trade-off between improved river condition and the cost of actions needed to achieve it (see Figure 2).
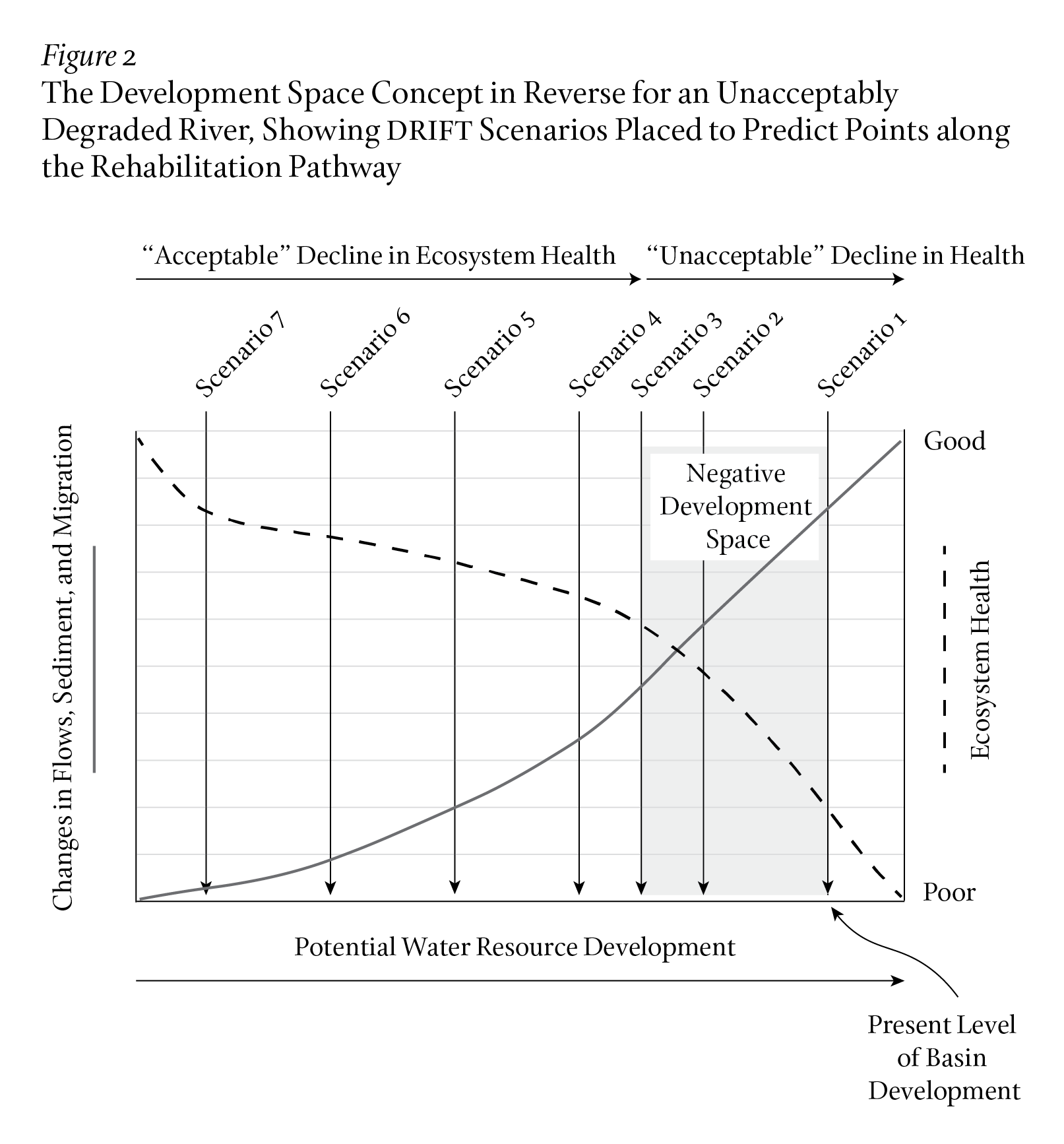
The process is in its infancy but, if the concept is to work, there is one caution. Having defined the end point of acceptable development, it should not be moved further to the right in later years as water demands increase. Instead, all innovation should be to the left of that line. In other words, sustainability requires that we should learn to live within the limits we set.
In the last few years, the concept has been discussed in stakeholder meetings and consultations between transboundary countries and it is now moving to being applied. The member states of the Cubango-Okavango River Basin in Southern Africa, for instance, are currently embarking on setting the boundaries of the Development Space for their shared basin.66 Their objective is to identify the point of greatest sustainable benefit, taking into account national development plans, the importance of the river system, and other relevant items.
Looking into the future, can the sustainability thinking and endeavor being developed by water scientists around the world guide us into a future in which the mindset changes from exploitation of inland waters to one of respect and caring management? In ways we can only begin to imagine, our future depends on this. The understanding is there; the skills are there; the technology is there; what to do and how to do it is known. What is not yet in place is a universal awareness and acceptance among governments, developers, and funders that this work can be done, and that it can help to open up the discourse on water developments leading to more equitable decisions. It can help countries plan for a sustainable future, moving from water grabs to wise stewardship, but only if there is the will to engage. Awareness is growing but it is almost literally one river at a time. Too often, the focus remains on the delivery of energy, food, and water, and the politics of water. As was written for the Darling River in Australia, “They did all the sums and added it all up, but they forgot about the river itself.”67 We could add for African rivers “and its people.” The same is true for all too many rivers on Earth.
A large amount of water resource development will continue in Africa for water and energy security. Large dams continue to be seen by many as the way forward, despite their enormous negative impacts on river systems and people. If we are to work in harmony with our planet, then tinkering with impact assessments of these developments at the level of individual projects no longer makes sense, as the scale of development is affecting whole river basins. Rather, such assessments should be obligatory, transparent, basin-wide, holistic ecological-social endeavors that are done before decisions are made. They should provide all stakeholder groups with the best possible information on the consequences of different management options, addressing all three pillars of sustainable development: ecological integrity, social equity, and economic wealth. Decision-makers should give all three equal weight in their considerations and not subsume them into an economic bottom line. Particularly in Africa, with its increasing population and traditional reliance on river resources, all uses of river systems should be brought into decisions, and the concept of no net harm adhered to. Future decision-makers should not need to say, “I did not know this could happen,” because they have the opportunity now to acquire a more balanced set of information to work with, and to be able to explore more deeply the implications of their decisions.
authors’ note
This essay is a contribution from the Africa’s Living Rivers Project, which is an initiative of the 2019 Stockholm Water Prize.
© 2021 by the American Academy of Arts & Sciences. Published under a CC BY-NC 4.0 license.