Game Changers from Outside the Nuclear Field
Nuclear energy makes up only part of the electricity mix, and factors that increase or decrease the attractiveness of one generating technology have consequences for the others. Thus, we should consider nuclear energy in context: as one choice among several for the electric sector.
The one policy development most likely to be a game changer for the electricity sector, and therefore nuclear energy, is policy action designed to mitigate the threat of global climate change. This action, whether in the form of subsidies, a carbon tax, or a cap-and-trade program, has the potential to drastically change the economics of energy use and generation. Here, we consider the possible effects such policies may have on nuclear power. We also consider two related game changers: the role of nuclear energy in a new “smart grid” and the potential rise of new technologies that could displace or compete with nuclear in a future energy mix.
Climate Change as a Game Changer
The threat of climate change has the potential to reshape the entire electricity sector. While the exact consequences of a rise in global temperatures remain the subject of debate and research, the basic science is clear: carbon dioxide is a greenhouse gas, and it is emitted in large quantities by the burning of fossil fuels. Since the Industrial Revolution, human generated greenhouse gas emissions have contributed to an increase in global surface temperatures. Many scientists believe that increasing the atmospheric concentration of carbon dioxide significantly higher than its preindustrial level of 260 to 280 parts per million by volume (ppmv) will lead to irreversible climate change. The current concentration stands at 390 ppmv and rising, presenting an urgent need to develop and implement low-emission sources of energy, particularly electricity, which uses a large and growing share of total primary energy. (Hereafter, emissions refers to the emission of greenhouse gases.)
This has significant ramifications for the nuclear energy industry. In the course of generating electricity, nuclear power plants emit no carbon dioxide, the primary greenhouse gas produced by humans, and the nuclear industry may benefit from policies designed to curb emissions growth. However, climate policy will not by itself lead to such a resurgence, and the role of nuclear power will depend on the form, strength, and implementation details of emissions-control mechanisms. Thus, aggressively moving forward on climate change can be seen as a necessary but not a sufficient condition for a large increase in nuclear power’s share of the worldwide electricity market.
The private sector is beginning to exhibit some interest in low-carbon technologies, such as nuclear energy, but only government action can change the incentive structure to make these technologies competitive with fossil fuels on a ten- to twenty-year timescale. The role of nuclear power in an emissions-constrained world will depend on several factors. First, an expansion of nuclear power is likely contingent on its efficacy in reducing emissions in a timely way, as compared to competing technologies. On the one hand, nuclear power is a tested and generally cost-competitive technology that generates no emissions during operation, while renewable technologies are not yet cost-competitive and have been tested only on relatively small scales. On the other hand, if financing, political, and safety concerns delay the construction of large-scale nuclear power plants, it may be preferable to rely on other technologies to make the necessary emissions cuts. Second, the role of nuclear power depends on its perceived efficacy in reducing emissions. The changing views of investors and the public toward nuclear power will help determine the willingness of utilities to construct expensive nuclear plants and of campaigners and regulators to include nuclear as a “green” technology. Third, prospects for nuclear power will depend heavily on the form of regulation adopted. How emissions controls are implemented will affect all players in the electricity sector, including nuclear power. A patchwork of differing local or state-level regulations is likely to produce different consequences from strong federal legislation, whether dictated by national policy, such as the now-defunct Waxman-Markey bill would have provided, or imposed in compliance with an international agreement. Additionally, the form such controls take will matter: direct subsidies for renewables, for instance, would likely diminish the prospects for nuclear, while nuclear power would prosper under cap-and-trade or direct carbon tax legislation. Finally, nuclear power plants provide a specific type of energy, namely, electricity. Nuclear, then, competes directly with other elements of the electric sector: coal, natural gas, and renewable sources like wind, solar, and tidal power. Thus, changes that affect any one of these technologies can significantly affect the others: for instance, a large decrease in the price of coal may render nuclear less attractive.
This state of affairs means that the fortunes of the nuclear industry are, in part, determined by both changing electricity demands and the competitiveness of other electricity-generating technologies. To assess whether climate change will be a game changer for nuclear, it is necessary to answer three questions. First, will states or the international community take action on the climate? Second, what form will this action take? And third, given this policy change, what is the role of nuclear?
What Will Make Governments Act? It has proven difficult to reach consensus on a global scale, but individual countries and regions have begun to take steps to reduce emissions. What could speed up this process, or increase the salience of climate change with voting populations? First, some weather-related catastrophe could possibly focus public and political attention on the problem of climate change. Climate change is likely to increase the frequency and severity of extreme weather events such as floods, droughts, hurricanes, and heat waves. Extreme events like the 2003 heat wave that killed thousands in Europe will become increasingly normal, as shown in Figure 4.
Figure 4: Small Increases in Mean Temperature Cause Many More Extreme Events Owing to Higher Temperatures and Their Consequences
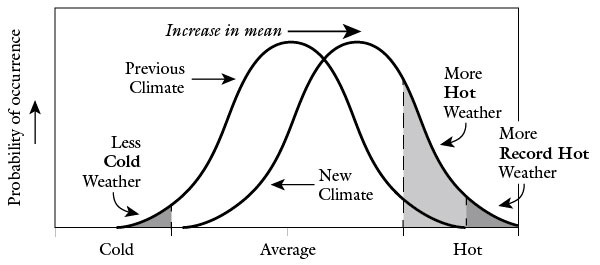
Source: Intergovernmental Panel on Climate Change (IPCC), Fourth Assessment Report, Box TS.5, Figure 1, “Schematic showing the effect on extreme temperatures when the mean temperature increases, for a normal temperature distribution.” Figure © IPCC 2007: WG1-AR4; used here with permission.
However, the prospect of a single event that is a game changer for climate policy is unlikely, unless that event is merely a trigger that occurs in a political environment already leaning toward taking action in the climate arena. There are two reasons why a single event is unlikely to stimulate policy change. One is simply a matter of scientific uncertainty: our incomplete understanding of certain natural phenomena complicates our ability to make specific predictions about the effects of climate change. Hurricane formation, in particular, depends on sea surface temperature, but also on a variety of other factors, including monsoon distribution, wind shear, El Niño/La Niña oscillations, and water vapor formation. The second serious problem is a matter of attribution: weather- and climate-related events occur against a natural backdrop of variability. While it may be possible to understand the altered probability distributions of these events in a warming world, it will never be possible to fully attribute any single event to climate change.
If single events are unlikely to prompt large-scale government action, perhaps specific regional predictions may compel policy-makers to act in order to mitigate threats to their territories or economic interests. Unfortunately, while such regional predictions can be made with a (currently modest) degree of assurance,40 it is difficult to provide decision-makers with precise information about the timescale and severity of the changes. Because greenhouse gases are well mixed in the atmosphere, and because so many components of atmosphere-ocean circulation are affected by large-scale processes, climate models are necessarily global. As a result, vast processing power is needed to run simulations, and global climate models operate on a coarsely resolved grid. Sub-grid scale processes are parametrized; that is, their effect is modeled from available data. As processing power improves, the resolution can be made finer, but no model can capture all the intricacies of the climate system at finely resolved spatial and temporal scales. Because of this uncertainty, regional climate change may not be viewed as an imminent and tangible threat meriting immediate response, but as a non-urgent global problem awaiting negotiated international solutions.
In the absence of a game-changing single event or set of predictions, economic considerations may contribute to a change in climate policy. The direction and magnitude of such change, however, remain uncertain. In the United States and China, an abundance of domestic coal makes it an inexpensive and secure source of electric power, at least if negative externalities are not taken into account. While other countries may not have vast domestic deposits of coal, it remains relatively easy and inexpensive to obtain on the international market. The sheer size and maturity of the coal industry means that it can often effectively resist change. Hydrocarbon resource constraints in general are unlikely to play a major role in traditional electricity generation over the timeline of this study: new discoveries of natural gas have increased known reserves by as much as a third, while petroleum is not heavily used for electricity in the developed world. The current economic situation in the developed world has also lowered demand for electricity and impeded the construction of new power plants. However, regulations at the state level in the United States have created growing markets for low-emission technologies, and demand for inexpensive “clean” energy is increasing abroad. Although attempts to recast climate legislation in terms of economic competitiveness or “green jobs” have met with mixed results, the bipartisan effort to defeat California’s Proposition 23, an attempt to repeal a statewide cap-and-trade system, successfully framed climate action as necessary to innovation and may mark a turning point. Outside the United States, China is aggressively developing renewable technology (in conjunction with ambitious fossil fuel and nuclear efforts), and European countries, particularly Germany, are poised to be major players in solar and wind energy. A desire to anticipate and compete in a future low-carbon world may lead more governments to incentivize clean-technology research and development, and would provide an economic impetus for emissions-control legislation.
Also unclear is whether binding international agreements among major emitters that would require domestic action to cut greenhouse gas emissions will come into force. Negotiations continue but have thus far been unsuccessful, with developing countries concerned about questions of fairness and the United States, in particular, reluctant or unable to commit to reduction targets. In several major countries, the priority given to climate change actions has dropped significantly in the past three years.41 These developments, coupled with the relative weakness of international institutions, make it difficult to imagine a viable and binding international agreement coming into force anytime soon. However, combinations of sustained grassroots pressure, political leadership, support from the business community, and economic incentives have resulted in a patchwork of different policies at national and subnational levels. The question for nuclear power is, how dependent is nuclear power on the likelihood and form of government action about climate change, at the local, national, and international levels?
What Form Could Climate Action Take? It is unclear how and if local policies will constitute a global emissions control regime, or what best practices may be adopted widely; still, it is instructive to examine common forms of regulation in order to determine the consequences for nuclear power. We can divide these policies into three rough categories. The simplest method involves taxing greenhouse gas emissions directly. A carbon tax has the advantage of addressing emissions while encouraging market-based development of new technology, and is a policy favored by many economists. Directly pricing carbon is likely, in the short term, to favor proven low-carbon base-load power technologies, of which nuclear is the primary example. Because of the long lifetimes of power plants, the consequences of these short-term decisions are likely to be favorable for nuclear power in the long term. In fact, a carbon tax could, at least in the United States, finally lead to the vaunted nuclear renaissance predicted by many experts.
Because of the prevalence of fossil fuels, a direct carbon tax is likely to increase electricity rates in the short term, provoking popular resistance. Even revenue-neutral taxes may leave their architects vulnerable to the attacks of political opponents. As a result, legislation in Europe and the United States often follows a cap-and-trade model, whereby the government issues a finite amount of permits to emit a certain substance. These permits can then be traded or sold, creating a market for emissions. Such policies have the advantage of determining a specific limit for emissions, while a tax on carbon may have to be adjusted several times to attain an emissions target. On the other hand, cap-and-trade regulations have proven difficult to design and even more so to implement. If the cap is set too high, then permits lose value; if too low, the price of energy can rise unacceptably. Allowing participants to purchase offsets—for example, through the “clean development mechanism” provided for in the Kyoto Protocol and practiced by some countries in the European Union—may lead to little or no net reduction in emissions from developed countries, and to reduced incentives to curb emissions in developing countries. The consequences for nuclear power in a cap-and-trade regime would therefore likely be similar to its growth under a carbon tax, but would depend strongly on the price and volatility of these carbon permits.
The third category of policies involves direct subsidies of low-emission technology. These subsidies come in several forms. Feed-in tariffs require utilities to purchase a set amount of electricity from renewable sources by guaranteeing a price for renewable energy over a specific time period, while renewable portfolio standards require utilities to supply a given percentage of total demand from designated renewable sources. While these approaches may facilitate the rapid introduction of otherwise prohibitively expensive technology, they do not necessarily encourage the development of new and more cost-competitive technologies. Additionally, policies of this type vary in the technologies they choose to subsidize because the definition of “renewable” or “low-emission” is open to interpretation. Is large-scale hydroelectric power, with its attendant environmental concerns, a “renewable” source of energy? Are biofuels, some of which promise to reduce emissions relative to gasoline but require vast land and water resources and also have poorly understood environmental effects, worthy of subsidy? Nuclear energy, in particular, falls into a gray area. Consider, for instance, that of the more than thirty U.S. states that have adopted renewable portfolio standards, only Ohio classifies nuclear technology as renewable. The consequences of these forms of subsidy for nuclear power will therefore depend on its classification and whether it is perceived as a clean technology.
What will be the Consequences of Government Climate Action for Nuclear Power? Such regulations have the potential to transform the entire energy sector, of which nuclear power is only a part. Game changers for other energy sources may thus be game changers for nuclear power by proxy. Changes in the electricity sector specifically will have the most direct impact on nuclear power. Game changers for petroleum, such as price shocks, drilling restrictions, or advances in refining technology, are unlikely to be direct game changers for nuclear power, at least in the developed world, where oil plays little to no role in electricity generation, unless electric vehicles achieve a high level of market penetration. In that case, demand for oil will be reduced, and the demand profile for electricity will change dramatically. Nuclear power will be affected by changes in the oil industry only insofar as those changes create incentives to electrify transport.
If a price is placed on carbon emissions, coal, the most common source of energy for electric power worldwide, will be the most affected, because coal-fueled power plants emit the most greenhouse gases per kilowatt-hour. Had the Waxman-Markey bill put before Congress in 2010 become law, the Environmental Protection Agency (EPA) price for carbon emissions permits would have ranged from $15 to $70 a ton, high enough to justify closing coal-fueled power plants. Carbon capture and sequestration (CCS) could in principle reduce or eliminate those emissions; a large research and development effort is devoted to studying that possibility. Many questions remain about the long-term stability and safety of geologic storage and the effects that a large sudden release of stored carbon dioxide would have on the climate. In addition, even if present techniques prove to be successful on a technical basis, CCS would lead to at least a 25 percent increase in cost and decrease in electrical output per ton of coal. Nevertheless, given the vast investment in coal plants around the world, retrofitting coal-fueled power plants with CCS, should it prove to be a safe and reliable technology, could become an attractive option (see Table 4).
Table 4: Life-Cycle Greenhouse Gas Emissions, per Gigawatt-Hour (GWh), for Various Sources of Energy
Energy Source | Tons CO2 Equivalent/GW |
Lignite | 1,000–1,400 |
Coal with Flue Gas Desulfurization | 800–1,100 |
Coal with Flue Gas Desulfurization and Carbon Capture and Sequestration | 150–200 |
Natural Gas Combined Cycle | 400–500 |
Natural Gas Combined Cycle with Carbon Capture and Sequestration | 200–250 |
Photovoltaic | Small–100 |
Hydro | Small–100 |
Biomass | Small–50 |
Wind Offshore | Small–30 |
Wind Onshore | Small–20 |
Nuclear | Small–50 |
1 GWh = 1 million KWh. Source: Adapted from Intergovernmental Panel on Climate Change, Fourth Assessment Report, vol. 3, “Mitigation of Climate Change” (2007).
With a price on carbon emissions, power plants fueled by natural gas gain an advantage over those fueled by coal. Gas combustion generates about half the CO2 emissions of coal combustion. Additionally, modern combined-cycle gas plants are much more efficient in terms of electricity generated for a given amount of fuel used than coal or nuclear plants, and gas plants are generally cheaper to build and license than nuclear plants. The overall levelized cost of a kilowatt-hour from a gas plant depends on the price of natural gas, but is generally competitive with coal and nuclear. However, the availability of gas depends on location; major investments are needed either for pipelines or for liquefied natural gas transport; care must be exercised to prevent leakage of the natural gas, itself a greenhouse gas; energy security concerns may make gas unattractive in certain areas (such as parts of Europe); and environmental concerns could curb shale-gas drilling techniques. Nevertheless, the discovery of new natural gas reserves in the United States could be a game changer for nuclear power and the electricity industry as a whole.
In an emissions-constrained world, the most direct competitors to nuclear energy are hydroelectric power and renewable energy sources such as wind, solar, and tide. These technologies also generate no carbon dioxide during operation, and they do not suffer from the negative perceptions attached to nuclear energy in the United States and elsewhere. However, the expansion of hydroelectric power is limited by both the availability of sites and environmental concerns. Moreover, renewables have potential drawbacks that could make nuclear power appear relatively attractive. First, generating large-scale power from wind, sun, or water requires a significant amount of surface area. Solar thermal, hydro, and biofuels also require large quantities of fresh water. Because renewables generate a small proportion of the world’s electricity, these concerns are relatively minor at present. However, as these sources begin to play a larger role in electricity generation, the larger scale may pose unexpected problems. Second, because wind farms and large solar installations tend to be located far from population centers, aggressively pursuing renewable sources means that, in many cases, new transmission lines will have to be built. While grid extension and modernization efforts are ongoing in developed countries and surging in China, large private and public investments are necessary. In addition, it is often difficult to obtain construction permits for high-voltage transmission lines. Barring significant changes at local, state, and national levels, the transmission problem will continue to impede renewable development, perhaps to the benefit of nuclear power. Finally, renewables provide intermittent power, and instantaneous generation is often difficult to predict owing to variable wind speeds, cloud cover, and wave heights. In the absence of reliable storage technologies, this sometimes unpredictable variability requires system operators to make special provision for integrating varying generation into the grid, and the proportion of total electricity that can be provided by these sources is limited. Nuclear, by contrast, operates at high capacity, providing a steady supply of constant power. The invention of inexpensive, viable, efficient storage mechanisms could greatly increase the appeal of renewable sources, perhaps to the detriment of nuclear power.
Nuclear Power and the Grid
Paradoxically, the base-load characteristic of nuclear power may put the technology at a competitive disadvantage in the future. This is because of potential changes in transmission and distribution that could be implemented to aid the integration of renewable sources. The development of the electricity sector in most low- and medium-income countries (and even some wealthy nations) is constrained by the capacity of the existing electric grid, and this will have consequences for nuclear power. Presently, the only way to achieve economies of scale for nuclear power is to utilize large capacity plants (above 500 MWe). Such large plants may not integrate well into existing electric grids, leading to a push for development of smaller modular reactors in an attempt to achieve economies of scale at the manufacturing level. Even smaller reactors, however, may be difficult to integrate into the “smart grids” of the future, which may be designed to handle the highly intermittent power generated by renewable sources. If renewables such as wind and solar are heavily subsidized, or if government standards mandate that a certain percentage of electricity must be generated by these technologies, it will be advantageous to utilize installed renewable capacity to its fullest potential. As renewable capacity increases, it is conceivable that the function of other technologies, such as nuclear, gas, and coal, will be to meet the residual demand not met by renewables.
Changes in Supply and Demand
The nature of electricity demand may change as well, particularly if large swaths of the transportation sector are electrified. If this occurs, power plants normally used for base-load may be forced to operate in load-following mode, delivering variable power to meet peaks and troughs in supply. This is technically possible, if complicated by the difficulty of quickly increasing or decreasing output and the need to prepare for unpredicted demand peaks. France, which depends on nuclear power for more than 80 percent of its electricity, operates some nuclear plants in load-following mode out of necessity. However, the economic case for nuclear plants is often based on their high capacity factors—over 90 percent for U.S. plants in 2009. This is because technologies that derive most of their levelized cost from amortizing initial capital investments, such as nuclear or hydro, are at a financial disadvantage in following a variable residual load compared to technologies that derive most of their levelized cost from fuel, such as gas. For the former technologies, one is paying the bigger share for time over which the money has been advanced and time keeps flowing by; for the latter, the bigger share of the cost is for gas, which can be turned off. If renewables are implemented on a large scale, residual demand may often drop below the capacity of existing nuclear plants: one study has found that German residual demand, for example, would drop below 20 GW more than fifty times a year by 2030, and would occasionally fall as low as zero. The economic justifications for nuclear power appear very different given these periods of low residual demand, and it is not clear that given all the attendant problems of a nuclear plant, utilities will be eager to invest in comparatively low-capacity plants.
In summary, the introduction of policies setting a significant price on emissions will favor all the low-emissions technologies: nuclear, renewables, and hydroelectric. Which will be most favored will depend on four major variables:
- Relative costs under the foreseen operating conditions, including land and water costs;
- Investment in and design choices for the electric grids (these two variables are highly interdependent);
- Both real and perceived environmental impact of the technologies; and
- Public sentiment as it evolves during deployment regarding all the technologies, but especially nuclear power.
The most obvious game changer for nuclear energy and for its competitors would be the development of a clean, safe, inexpensive, and widely deployable technology for electricity generation. Fusion fits the bill, but despite the scientific advances discussed above, it is unlikely to be widely commercially available in the timescale we consider. The list of potential disruptive technologies is long, and categorizing specific possible advances is beyond the scope of this paper. However, any game-changing technology must address the shortcomings of current technologies: the waste, expense, and proliferation concerns of nuclear, and the intermittency, high cost, or resource constraints associated with renewables. It requires no special understanding of current research and development to appreciate these problems, and to anticipate technologies that may solve them.
ENDNOTES