Chapter 3: Lying Outside the Laboratory: The Impact of Imagery and Emotion on the Neural Circuitry of Lie Detection
Elizabeth A. Phelps
One of the challenges of research on lie detection is the difference between instructed lying in a laboratory setting and the types of situations one might encounter outside the laboratory that would require the use of lie detection techniques. The development of techniques for lie detection is based on laboratory studies of lying. However, the characteristics of lies outside the laboratory may differ in important ways. For instance, if someone is accused of a crime, there are two possible scenarios. First, the person may be innocent. If this is the case, he or she is likely upset and wondering how the accusers could think the charges are plausible. Given the serious circumstances, this person might ruminate on this last point and be quite concerned about proving his or her innocence. On the other hand, if a person is accused of a crime he or she actually committed, there might be an anxious or guilty feeling and an effort to formulate a false alibi. This person might think about this lie in detail and elaborate on the lie in an effort to be especially convincing when asked about the alibi. In both cases, the person accused of the crime faces a highly emotional situation and has ample opportunity to mentally image the circumstances that occurred and ruminate on the situation. It is these factors, emotion and imagery, that differ between the real lie and the laboratory lie. Research in cognitive neuroscience has shown that both imagery and emotion can alter the representation of events. Given that lies outside the laboratory are likely to be personally relevant and emotional, and also imagined with elaboration and repetition, any successful lie detection techniques will need to consider these factors. In this essay, I will explore some of the ways imagery and emotion might impact the potential neural signatures of lying.
There are two possible uses of functional magnetic resonance imaging (fMRI) for lie detection. One is to assess familiarity. Imagine that the police want to know whether a suspect has been to the scene of a crime. The suspect might deny any familiarity with that location. In using fMRI to assess familiarity, the police could show the suspect a picture of the scene and look for a pattern of blood oxygenation level dependent (BOLD) signal in his or her brain that indicates previous experience with that scene. In this case, the use of fMRI for lie detection is based on our knowledge about the neural representation of memory. The second use of fMRI for lie detection is to detect deception. One would expect that the neural systems involved when someone is lying or telling the truth are different. The assumption underlying lie detection techniques for detecting deception is that the truth is the natural response. When individuals lie they have to inhibit the truth to generate the lie. In this case, lying results in conflict between the truth and the lie. The use of fMRI to detect deception is based on our knowledge about the neural representation of conflict. When lying outside the laboratory, the stakes are high, the individual is highly emotional, and the story is practiced, rehearsed, or imagined. Because of this, the use of fMRI for lie detection will need to consider how imagery and emotion might alter the neural representation of memory and conflict.
To address these questions, I will first review what is known about the neural signatures of using fMRI to detect familiarity. Have we identified reliable neural markers for item or event familiarity? In other words, when presented with a face or scene seen before or that is reminiscent, does the brain respond with a pattern of activity that is different in ways that can be measured with fMRI? This question was addressed in a recent study by Gonsalves, Wagner, and colleagues (2005). In this study, the participants were presented with a series of faces. Afterwards, they were given a recognition test in which some of the faces were presented earlier, some were morphed to look somewhat like the faces presented earlier, and others were novel. For each face, participants were asked to judge whether they recollected having seen the face earlier, if it seemed familiar, or if it was new. There were a few regions in the temporal lobe, the hippocampal cortex and fusiform gyrus, where BOLD responses differed depending on the mnemonic judgment. The term hippocampal cortex refers to a collection of regions known to be important for memory, including the hippocampus proper and regions around and underneath it, such as the parahippocampal cortex. These regions showed more activity when the faces were judged to be less familiar than when they were more familiar. The fusiform gyrus, which plays an important role in processing faces, showed more activity when faces were more familiar. These results suggest the possibility of a neural signature for familiarity that could be detected with fMRI (see Figure 1).
However, what do we know about how responses in the hippocampus, parahippocampus, and fusiforn gyrus might be altered with imagery and emotion? First, let’s explore the role of imagery. A classic paradigm demonstrates the importance of imagery in memory. Imagine you were presented the following list of words: SOUR, CANDY, SUGAR, BITTER, GOOD, TASTE, TOOTH, NICE, HONEY, SODA, CHOCOLATE, HEART, CAKE, TART, PIE. After the presentation of this list and a short delay you are given a recognition test. For instance, you might be asked if tart was on the list, in which case you would say “yes.” If you were asked if chair was on the list, you would correctly respond “no.” However, what if you were asked if sweet was on the list? If you go back a few sentences, you will see that sweet was not on the list, however most participants in experiments like this say “yes” it was on the original list. This is because sweet is strongly associated with all the words on the list. Even though sweet was not on the list, your mind most likely came up with the image of sweet when you were reading the words. This type of mistake is often called a false memory, but is not really a false memory. We have memories both for images that we internally generate and events that occur in the external world. In this case, the word sweet is really an imagined memory, something that was generated when you saw the initial list of words, and therefore, most people misremember actually having seen it before. This is an example of mental imagery creating a memory that results in a memory mistake.
Can fMRI images of the brain distinguish imagined or false memories from memories based on perceptual experience? As the study by Gonsalves and colleagues (2005) demonstrates, fMRI signals can indicate familiarity, but what happens when an item is familiar because the individual has imagined it? Using the word list paradigm described above it was shown that some regions known to be important for memory, such as the hippocampus, do not differentiate memories for events that are perceptually experienced from events that are imagined (Cabeza et al., 2001). In other words, our memories for real and imagined events rely on overlapping neural systems. If we were to look at responses in the hippocampus, we could not differentiate if an event is familiar due to perceptual experience or mental imagery. However, there are other regions of the hippocampal cortex, specifically the parahippocampus, that are more important in processing the perceptual details of memory. In this same study, this region showed greater activation to true relative to false memories. This study, and others like it (see Schacter and Slotnick, 2004 for a review), indicates that for many brain regions known to be important in memory, such as the hippocampus, it does not matter whether an experienced event was the result of our thought or our perception. Given this, we cannot look at these memory-related brain regions to reveal whether a person is remembering accurately. Other brain regions, such as the parahippocampus and fusiform gyrus, are more specifically involved in perceptual processing and memory for perceptual details (Schacter and Slotnick, 2004). These regions may provide a signal of familiarity for scenes and faces.
However, one difficulty in relying on BOLD signal responses in regions involved in perceptual aspects of memory, such as the parahippocampus or fusiform gyrus, to judge whether a suspected criminal is familiar with a scene or face is that perception is often altered by emotion. For the criminal whose brain is being imaged to judge involvement in a crime, pictures of the scene of the crime or partners in crime are likely highly emotional. Changes in perception occur with emotion, and research has demonstrated changes in BOLD signal in both the parahippocampus and fusiform gyrus for emotional scenes and faces. For example, a study looking at individuals remembering 9/11 found that people who were closer to the World Trade Center showed less activity in the parahippocampus when recalling the events of 9/11 than when recalling less emotional events (Sharot et al., 2007). As indicated earlier, the parahippocampus also shows less activation when a face is more familiar (Gonsalves et al., 2005). Even though imaging this region might reveal perceptual qualities of memory, this region might also be influenced by emotion. If the event is highly emotional, signals in the parahippocampus might not be a reliable indicator of familiarity.
What about the fusiform gyrus? This region is known for processing faces (Kanwisher and Yovel, 2006, for a review). As indicated by Gonslaves and colleagues (2005) this region also shows stronger activation for more familiar faces. However, emotion also influences responses in the fusiform gyrus, so that in a highly emotional situation the signal from this region might be somewhat altered. For faces that are equally unfamiliar, more activation is observed in the fusiform gyrus for faces with fear expressions (Vuilleumier et al., 2001). Furthermore, the face itself does not need to be fearful. If the context in which the face is presented is fearful, greater activation of the fusiform gyrus is observed (Kim et al., 2004). Because of this, responses in this region may not be a reliable indicator of familiarity with a face in an emotional situation.
When researchers look at the brain’s memory circuitry to detect familiarity with a scene or person, for some regions it may be difficult to differentiate events that a person imagined, rehearsed, or thought were plausible from those that actually occurred. Memories are formed for events that happen only in our minds and events that happen in the outside world. The regions that are important in the perceptual aspects of memory are influenced by emotion, so they might not be good detectors of familiarity if the situation is emotional. In other words, the imagery and emotion that would likely be present when a lie is personally relevant and important might interfere with the use of fMRI signals to detect familiarity.
The second potential use of fMRI for lie detection relies on our knowledge of the neural circuitry of conflict. It is assumed that lying results in a conflict between the truth and the lie. How might emotion and imagery influence the neural signatures of conflict? Two regions that have been highlighted for their role in responding to conflict or interference are the anterior cingulate cortex and the inferior frontal gyrus. These regions have also been implicated in studies of lie detection (e.g., Kozel et al., 2004; Langelben et al., 2005). One classic task used to detect conflict-related responses is the Stroop test, in which participants are shown a list of words and asked not to read the words but to name the colors in which the words are printed. For instance, if the word table is presented in blue ink, participants are asked to say “blue.” Most of the time, participants can fairly easily ignore the words and name the color of the ink. However, if the words the participants are asked to ignore are the names of colors, it is much more difficult. For example, it typically takes significantly longer for participants to name the ink color “blue” if the word they are asked to ignore is red as opposed to table. This longer reaction time is due to the conflict between reading the word red and saying the word “blue.” Naming the color of ink for color words in comparison to other words results in significant activation of an anterior, dorsal region of the cingulate cortex (Carter and van Veen, 2007) and this same region shows activation in many laboratory studies of lie detection (e.g., Kozel et al., 2004).
However, difficulty in naming the ink color of words is not only slower for color words. In a variation of the Stroop task, called the emotional Stroop task, subjects are presented with highly emotional words printed in different colors of ink. When asked to ignore the words and name the ink color, it takes significantly longer to name the color for emotional words in comparison to neutral words. Interestingly, emotional variations of the Stroop task also result in activation of the anterior cingulate, but a slightly different region that is more ventral than that observed in the classic Stroop paradigm (Whalen et al., 1998). In a meta-analysis of a number of conflict tasks, Bush et al. (2000) confirmed this division within the anterior cingulate (see Figure 2). Cognitive conflict tasks, such as the classic Stroop task, typically result in activation of the dorsal anterior cingulate, whereas emotional conflict tasks, as demonstrated by the emotional Stroop task, result in activation of the ventral anterior cingulate. This suggests that this specific neural indicator of conflict is significantly altered depending on the emotional nature of the conflict.
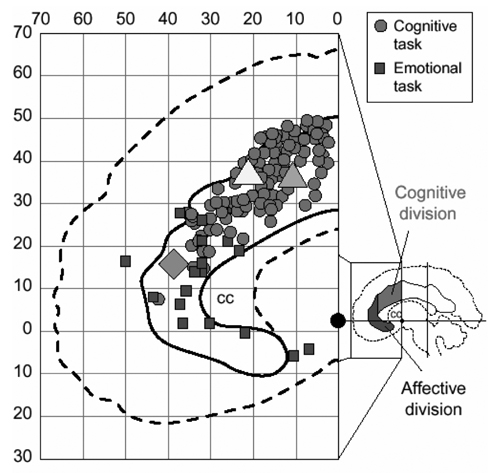
Figure 2. Meta-analysis of fMRI studies showing anterior cingulate activation for cognitive (circles) and emotional (squares) tasks demonstrating a cognitive-affective division within the anterior cingulate. Consistent with this division, cognitive (triangles) and affective (diamond) versions of a Stroop task results in activation of dorsal and ventral regions of the anterior cingulate, respectively. Reprinted with permission from Bush et al., 2000.
Another region often implicated in conflict or interference in studies of lie detection is the inferior frontal gyrus. In fact, some studies of lie detection have suggested that activation of this region is the best predictor of whether a participant is lying (Langleben et al., 2005). The role this region plays in conflict or interference monitoring has traditionally been examined with the Sternberg Proactive Interference paradigm. In a typical version of this paradigm, a participant is shown a set of stimuli and told to remember it. For example, the set might include three letters, such as B, D, F. After a short delay the participant is presented a letter and asked, “Was this letter in the target set?” If the letter is D, the participant should answer “yes.” In the next trial the participant is given another target set, such as K, E, H.
At this point, if the participant is shown the letter P, she or he should say “no.” If the participant is shown the letter B, the correct answer is also “no.” However, for most participants it will take longer to correctly respond “no” to B than P. This is because B was a member of the immediately preceding target set (B, D, F), but it is not a member of the current target set (K, E, H). On the preceding trial, a minute or so earlier, the participant was ready to respond “yes” to B. To correctly respond “no” to B on the current trial requires the participant to inhibit this potential “yes” response and focus only on the current target set. This requirement for inhibition is not necessary if the probe letter is P, which was not a member of either the preceding or current target set. Research using both brain imaging (D’Esposito et al., 1999; Jonides and Nee, 2006) and lesion (Thompson-Schill et al., 2002) techniques has shown that the inferior frontal gyrus plays an important role in resolving this type of interference or conflict. It is believed this region might be linked to lying because in order to lie one must inhibit the truth, which creates conflict or interference (e.g., Langleben et al., 2005).
In order to examine the impact of emotion on this type of interference, a recent study used a variation of the typical Sternberg Proactive Interference paradigm in which the stimuli were emotional words or scenes, instead of letters or neutral words and scenes. An examination of reaction times found that the inhibition of emotional stimuli was faster than neutral stimuli, suggesting that emotion can impact processing in this interference paradigm (Levens and Phelps, 2008). Using fMRI to examine the neural circuitry underlying the impact of emotion on the Sternberg Proactive Interference para-digm revealed that the inhibition of emotional stimuli on this task engages a slightly different network, including regions of the anterior insula cortex and orbitofrontal cortex (Levens et al., 2006). Much like the results observed with the anterior cingulate, this suggests that emotion alters the neural circuitry of inhibition or conflict observed in the inferior frontal gyrus. Although further studies are needed to clarify the impact of emotion on interference resolution mediated by the inferior frontal gyrus, these initial results suggest that this neural indicator of conflict in lying may also be different in highly emotional situations.
There is abundant evidence that emotion can influence the neural circuitry of conflict or interference identified in laboratory studies of lie detection, but can imagery or repetition also alter responses in these regions? It seems possible that practicing a lie repeatedly, as one might after generating a false alibi, could reduce the conflict experienced when telling that lie. If this is the case, we might expect less evidence of conflict with practice or repetition. This finding has been observed with the classic Stroop paradigm. A number of behavioral studies have demonstrated that practice can diminish the Stroop effect (see MacLeod, 1991, for a review). It has also been shown that practicing the Stroop task significantly reduces conflict-related activation in the anterior cingulate (Milhan et al., 2003). To date, there is little research examining how imagery might alter the neural circuitry of conflict or interference as represented in the inferior frontal gyrus, but these findings suggest that at least some neural indicators of conflict or interference may be unreliable if the task (or lie) is imagined, practiced, and rehearsed.
Although the use of fMRI to detect lying in legal settings holds some promise, there are some specific challenges in developing these techniques that have yet to be addressed. Out of necessity, the development of techniques for lie detection relies on controlled laboratory studies of lying. However, lying in legally relevant circumstances is rarely so controlled. This difference should be kept in mind when building a neurocircuitry of lie detection that is based on unimportant lies told by paid participants in the laboratory, but is intended to be applied in legally important situations to people outside the laboratory facing far higher stakes. Because of this, it is important to examine exactly what might differ between the laboratory lie and the other lies that could impact the usefulness of these techniques. In this essay, I have explored two factors–imagery and emotion–and highlighted how research suggests that the neural signatures identified in current fMRI lie detection technologies might be quite different in their utility when the lies detected are not generated in the laboratory. This problem of applying laboratory findings to other, more everyday and/or personally relevant and important circumstances is a challenge for all studies of human behavior. However, addressing this challenge becomes especially critical when we attempt to use our laboratory findings to generate techniques that can potentially impact individuals’ legal rights. Until this challenge can be addressed, the use of fMRI for lie detection should remain a research topic, instead of a legal tool.
REFERENCES
Bush, G., P. Luu, and M.I. Posner. 2000. Cognitive and emotional influences in anterior cingulate cortex. Trends in Cognitive Science 4:215–222.
Cabeza, R., S.M. Rao, A.D. Wagner, A.R. Mayer, and D.L. Schacter. 2001. Can medial temporal lobe regions distinguish true from false? An event-related functional MRI study of veridical and illusory recognition memory. Proceedings of the National Academy of Sciences 98:4805–4810.
Carter, C.S., and V. van Veen. 2007. Anterior cingulate cortex and conflict detection: an update of theory and data. Cognitive, Affective, Behavioral Neuroscience 7:367–379.
D’Esposito, M., B.R. Postle, J. Jonides, and E.E. Smith. 1999. The neural substrate and temporal dynamics of interference effects in working memory as revealed by event-related functional MRI. Proceedings of the National Academy of Sciences 96:7514–7519.
Gonsalves, B.D., I. Kahn, T. Curran, K.A. Norman, and A.D. Wagner. 2005. Memory strength and repetition suppression: Multimodal imaging and medial temporal cortical contributions to recognition. Neuron 47:751–781.
Jonides, J., and D.E. Nee. 2006. Brain mechanisms of proactive interference in working memory. Neuroscience 139:181–193.
Kanwisher, N., and G. Yovel. 2006. The fusiform face area: a cortical region specialized for the perception of faces. Philosophical Transactions of the Royal Society: B Biological Science 361:2109–2128.
Kim, H., L.H. Somerville, T. Johnstone, S. Polis, A.L. Alexander, L.M. Shin, and P.J. Whalen. 2004. Contextual modulation of amygdala responsivity to surprised faces. Journal of Cognitive Neuroscience 16:1730–1745.
Kozel, F.A., T.M. Padgett, and M.S. George. 2004. A replication study of the neural correlates of deception. Behavioral Neuroscience 118:852–856.
Langleben, D.D., J.W. Loughead, W.B. Bilker, K. Ruparel, A.R. Childress, S.I. Busch, and R.C. Gur. 2005. Telling truth from lie in individual subjects with fast event-related fMRI. Human Brain Mapping 26:262–272.
Levens, S.M., M. Saintilus, and E.A. Phelps. 2006. Prefrontal cortex mechanisms underlying the interaction between emotion and inhibitory processes in working memory. Cognitive Neuroscience Society, San Francisco, CA.
Levens, S.M., and E.A. Phelps. 2008. Emotion processing effects on interference resolution in working memory. Emotion 8:267–280.
MacLeod, C.M. 1991. Half a century of research on the Stroop effect: An integrative review. Psychological Bulletin 109:163–203.
Milhan, M.P., M.T. Banich, E.D. Claus, and N.J. Cohen. 2003. Practice-related effects demonstrate complementary roles of anterior cingulate and prefrontal cortices in attentional control. Neuroimage 18:483–493.
Schacter, D.L., and S.D. Slotnick. 2004. The cognitive neuroscience of memory distortion. Neuron 44:149–160.
Sharot, T., E.A. Martorella, M.R. Delgado, and E.A. Phelps. 2007. How personal experience modulates the neural circuitry of memories of September 11. Proceedings of the National Academy of Sciences 104:389–394.
Thompson-Schill, S.L., J. Jonides, C. Marshuetz, E.E. Smith, M. D’Esposito, I.P. Kan, R.T. Knight, and D. Swick. 2002. Effects of frontal lobe damage on interference effects in working memory. Cognitive, Affective, Behavioral Neuroscience 2:109–120.
Vuilleumier, P., J.L. Armony, J. Driver, and R.J. Dolan. 2001. Effects of attention and emotion on face processing in the human brain: an event-related fMRI study. Neuron 30:829–841.
Whalen, P.J., G. Bush, R.J. McNally, S. Wilhelm, S.C. McInerney, M.A. Jenike, and S.L. Rauch. 1998. The emotional counting Stroop paradigm: a functional magnetic resonance imaging probe of the anterior cingulate affective division. Biological Psychiatry 44:1219–1228.